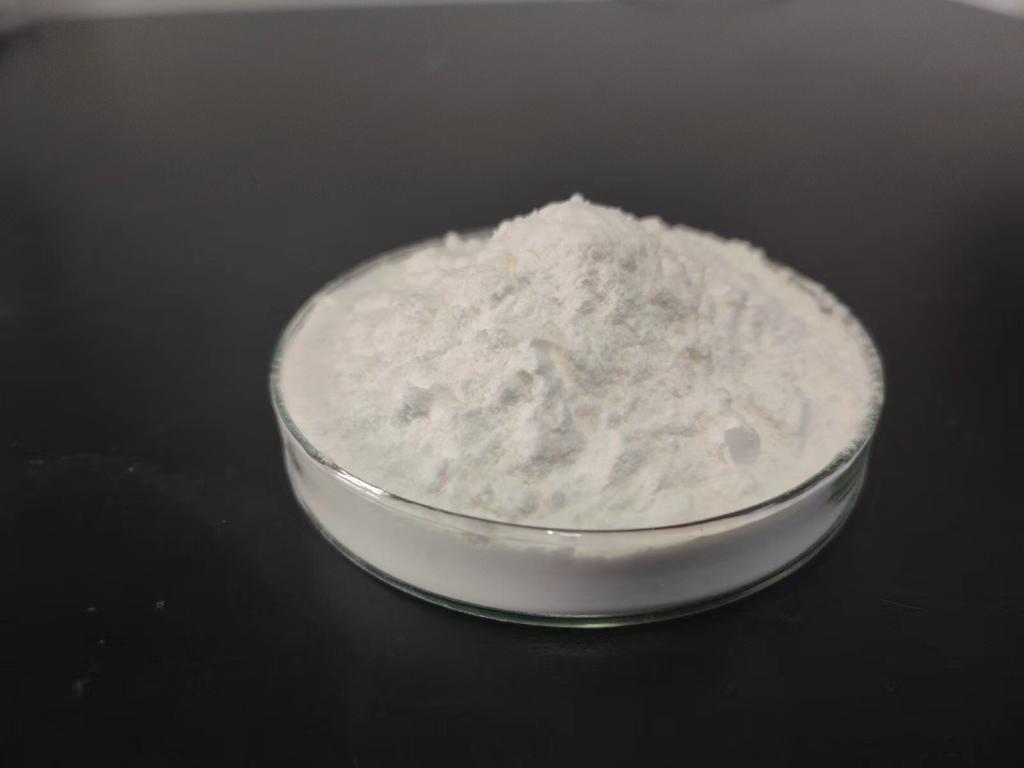
ε-Polylysine hydrochloride (ε-PL) is a naturally occurring, biodegradable polymer known for its antimicrobial properties. It is widely used in various industries, including food preservation, biomedical applications, and pharmaceuticals. As the demand for sustainable and environmentally friendly materials increases, it is crucial to understand the environmental impact of ε-PL. This article aims to comprehensively explore the environmental implications of ε-PL, from its production and usage to its degradation and disposal.
Overview of ε-Polylysine Hydrochloride
Chemical Structure and Properties
ε-Polylysine is a homopolymer composed of L-lysine residues linked by peptide bonds between the ε-amino group and the α-carboxyl group. The hydrochloride form enhances its solubility and stability in aqueous solutions. Key properties of ε-PL include:
Biodegradability: ε-PL can be enzymatically degraded into lysine, an essential amino acid.
Antimicrobial Activity: It is effective against a broad spectrum of microorganisms.
Biocompatibility: ε-PL is non-toxic and safe for various applications.
Production of ε-Polylysine
ε-PL is primarily produced through microbial fermentation using strains of Streptomyces albulus. The fermentation process involves the cultivation of bacteria in nutrient-rich media, followed by the extraction and purification of ε-PL.
Fermentation: Microbial fermentation is considered an environmentally friendly production method compared to chemical synthesis. It uses renewable resources and generates minimal waste.
Extraction and Purification: The purification process typically involves several steps, including precipitation, filtration, and chromatography. These steps require solvents and energy, contributing to the overall environmental footprint.
Environmental Impact of Production
Resource Utilization
The production of ε-PL involves the use of various resources, including raw materials, water, and energy.
Raw Materials: The primary raw materials for ε-PL production are sugars and amino acids used as substrates for microbial fermentation. The use of renewable agricultural products as substrates is a positive aspect, reducing dependence on fossil fuels.
Water Consumption: Fermentation processes require significant amounts of water for microbial growth, cooling, and purification. Efficient water management practices are essential to minimize the environmental impact.
Energy Consumption: The energy required for maintaining fermentation conditions, as well as for downstream processing, can contribute to the carbon footprint. Utilizing renewable energy sources can mitigate this impact.
Emissions and Waste
The environmental impact of ε-PL production also includes emissions and waste generated during the process.
Greenhouse Gas Emissions: Fermentation processes can produce carbon dioxide as a byproduct. The overall greenhouse gas emissions depend on the energy sources used and the efficiency of the process.
Solid and Liquid Waste: The extraction and purification stages generate solid and liquid waste, including spent biomass and solvents. Proper waste management practices, such as recycling and safe disposal, are crucial to minimize environmental harm.
Environmental Impact of Usage
Applications and Benefits
ε-PL is used in various applications, each with its environmental implications.
Food Preservation: ε-PL is widely used as a natural preservative in food products. Its use can extend the shelf life of food, reducing food waste and the environmental burden associated with food production and disposal.
Biomedical Applications: In medical devices and coatings, ε-PL's antimicrobial properties can prevent infections, leading to better health outcomes and potentially reducing the need for antibiotic treatments.
Pharmaceuticals: ε-PL is used in drug delivery systems, enhancing the efficacy of medications and reducing the frequency of dosing. This can lead to more efficient use of pharmaceuticals and less waste.
Human Health and Safety
The use of ε-PL in various applications must also consider potential impacts on human health and safety.
Biocompatibility: ε-PL is generally regarded as safe for human use, with low toxicity and minimal side effects. Its degradation into lysine further supports its safety profile.
Antimicrobial Resistance: The widespread use of antimicrobial agents, including ε-PL, raises concerns about the development of antimicrobial resistance. Responsible use and continuous monitoring are essential to mitigate this risk.
Degradation and Disposal
Biodegradability
One of the key environmental benefits of ε-PL is its biodegradability. ε-PL can be broken down by enzymes into lysine, which is then further metabolized by microorganisms.
Enzymatic Degradation: Specific enzymes can cleave the ε-polylysine chain into lysine residues. This process occurs naturally in the environment, facilitated by microbial activity.
Environmental Decomposition: ε-PL decomposes into harmless byproducts that do not persist in the environment, reducing the risk of long-term pollution.
Waste Management
The disposal of ε-PL-containing products and waste generated during production must be managed properly to minimize environmental impact.
Recycling and Reuse: Wherever possible, ε-PL-containing materials should be recycled or reused to extend their life cycle and reduce waste.
Safe Disposal: Waste materials, including spent fermentation biomass and purification byproducts, should be disposed of in accordance with environmental regulations to prevent contamination.
Life Cycle Assessment
A comprehensive life cycle assessment (LCA) can provide valuable insights into the overall environmental impact of ε-PL. An LCA considers all stages of the product life cycle, from raw material extraction and production to usage and disposal.
Goal and Scope Definition: The first step in an LCA is to define the goal and scope, including the system boundaries and functional unit.
Inventory Analysis: This involves collecting data on all inputs and outputs associated with each stage of the life cycle, including raw materials, energy, water, emissions, and waste.
Impact Assessment: The inventory data is then analyzed to assess the environmental impacts, such as global warming potential, water use, and ecological footprint.
Interpretation: The results are interpreted to identify areas for improvement and to inform decision-making for sustainable practices.
Key Findings from LCA Studies
Preliminary LCA studies on ε-PL production and usage suggest that:
Renewable Substrates: Using renewable agricultural substrates for fermentation can significantly reduce the environmental impact.
Energy Efficiency: Improving energy efficiency and utilizing renewable energy sources can lower greenhouse gas emissions.
Waste Management: Effective waste management practices, including recycling and safe disposal, are crucial for minimizing environmental harm.
Sustainable Practices and Future Directions
Optimization of Production Processes
Continued research and development efforts are needed to optimize the production processes of ε-PL, focusing on:
Fermentation Efficiency: Enhancing the efficiency of microbial fermentation can reduce resource consumption and waste generation.
Energy Use: Integrating renewable energy sources and improving process energy efficiency can lower the carbon footprint.
Water Conservation: Implementing water recycling and conservation measures can reduce water consumption.
Development of Eco-Friendly Applications
Exploring new applications of ε-PL that align with sustainability goals can further enhance its environmental benefits.
Biodegradable Packaging: ε-PL can be used in biodegradable packaging materials, providing an alternative to conventional plastics and reducing plastic pollution.
Sustainable Agriculture: ε-PL-based formulations can be developed for use in sustainable agriculture practices, such as natural pesticides or soil conditioners.
Policy and Regulatory Considerations
Ensuring the sustainable use of ε-PL requires supportive policies and regulatory frameworks.
Incentives for Sustainable Production: Governments can provide incentives for companies to adopt sustainable production practices for ε-PL.
Regulatory Standards: Establishing clear regulatory standards for the use and disposal of ε-PL-containing products can ensure environmental protection.
Monitoring and Reporting: Implementing monitoring and reporting requirements can help track the environmental impact of ε-PL and promote transparency.
Conclusion
ε-Polylysine hydrochloride is a versatile and environmentally friendly material with significant potential in various applications, from food preservation to biomedical coatings. Its biodegradability and biocompatibility make it a promising candidate for sustainable development. However, understanding and mitigating its environmental impact requires a comprehensive approach, including optimizing production processes, developing eco-friendly applications, and implementing supportive policies and regulations. By embracing these strategies, the benefits of ε-PL can be maximized while minimizing its environmental footprint, contributing to a more sustainable future.