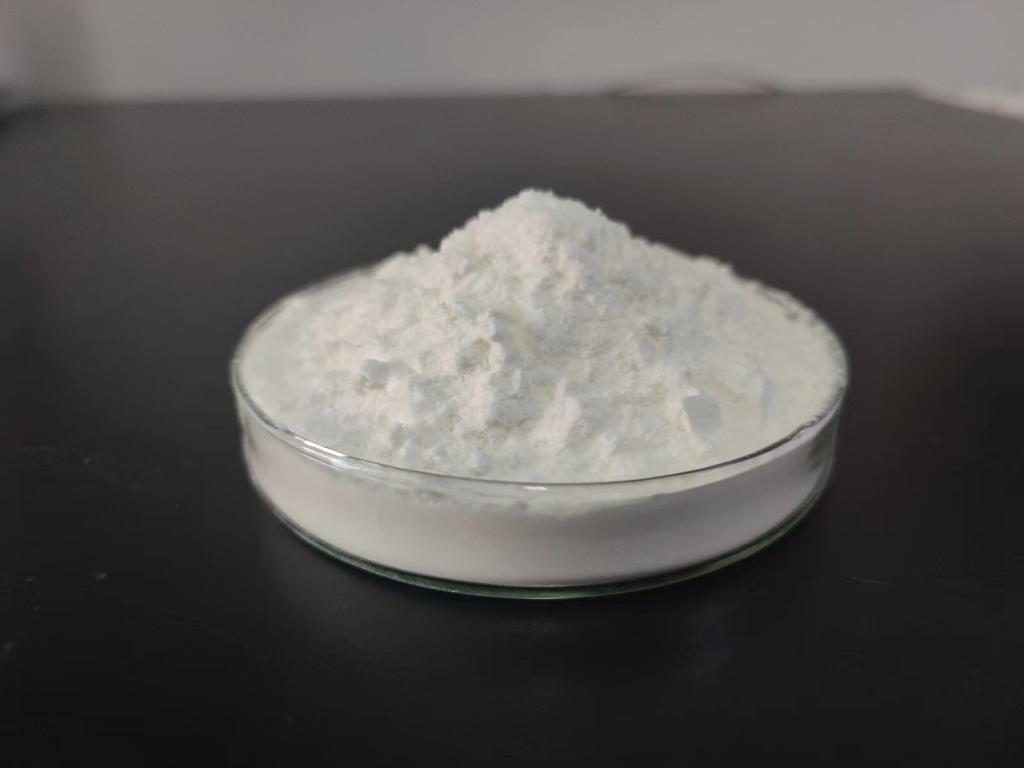
ε-Polylysine hydrochloride (ε-PL) is a naturally occurring biopolymer known for its broad-spectrum antimicrobial activity, biocompatibility, and biodegradability. Initially recognized for its use as a food preservative, ε-PL has garnered significant interest in biomedical and pharmaceutical applications, particularly in enhancing drug delivery systems. This article explores the mechanisms of action of ε-PL, examining its antimicrobial properties, interaction with cell membranes, and potential applications in various fields. Understanding these mechanisms is crucial for leveraging ε-PL's full potential in scientific and industrial applications.
Chemical Structure and Properties
Composition and Structure
ε-Polylysine is a homopolymer composed of L-lysine residues linked by peptide bonds between the ε-amino group and the α-carboxyl group of adjacent lysine molecules. Typically, ε-PL contains 25-35 lysine residues, forming a positively charged, linear chain. The hydrochloride form, ε-polylysine hydrochloride, enhances its solubility and stability in aqueous solutions.
Physicochemical Properties
Key properties of ε-PL include:
Positive Charge: The polymer's cationic nature facilitates interactions with negatively charged molecules and surfaces.
Water Solubility: ε-PL hydrochloride is highly soluble in water, making it easy to incorporate into various formulations.
Biodegradability: ε-PL can be enzymatically degraded into lysine, an essential amino acid, ensuring minimal environmental impact and biocompatibility.
Antimicrobial Mechanisms
Interaction with Bacterial Cell Membranes
The primary mechanism of ε-PL's antimicrobial activity involves its interaction with bacterial cell membranes. The positively charged ε-PL binds to the negatively charged components of bacterial cell membranes, such as phospholipids and lipopolysaccharides. This interaction disrupts the integrity of the membrane, leading to increased permeability, leakage of intracellular contents, and eventual cell death.
Disruption of Membrane Integrity
Electrostatic Interaction: The cationic ε-PL molecules are attracted to the anionic cell membrane components, leading to strong electrostatic interactions.
Membrane Permeabilization: ε-PL inserts into the lipid bilayer, causing physical disruptions and creating pores in the membrane.
Leakage of Cellular Contents: The formation of pores results in the leakage of essential intracellular components, such as ions, nucleotides, and metabolic intermediates, compromising cell viability.
Inhibition of Enzymatic Activities
ε-PL can also inhibit essential enzymatic activities within microbial cells. By binding to enzymes involved in vital cellular processes, ε-PL disrupts their normal functions, further contributing to the antimicrobial effect.
Inhibition of Cell Wall Synthesis: ε-PL interferes with the synthesis of peptidoglycan, a crucial component of bacterial cell walls, leading to weakened cell structure and increased susceptibility to osmotic pressure.
Disruption of Metabolic Pathways: By binding to key enzymes in metabolic pathways, ε-PL hampers the production of energy and essential biomolecules, ultimately leading to cell death.
Synergistic Effects with Other Antimicrobials
ε-PL can enhance the efficacy of other antimicrobial agents through synergistic interactions. When used in combination with antibiotics or other antimicrobial compounds, ε-PL can increase membrane permeability, facilitating the entry of these agents into the bacterial cell and potentiating their effects.
Mechanisms in Drug Delivery Systems
Enhanced Cellular Uptake
The cationic nature of ε-PL facilitates its interaction with the negatively charged cell membranes, enhancing the cellular uptake of ε-PL-based drug delivery systems. This property is particularly useful for delivering therapeutic agents that require efficient intracellular delivery.
Endocytosis: ε-PL-based nanoparticles and complexes can be readily internalized by cells through endocytosis, increasing the intracellular concentration of the therapeutic agent.
Membrane Penetration: ε-PL can facilitate the direct penetration of drugs across the cell membrane, bypassing the need for endocytic pathways and improving delivery efficiency.
Controlled Release of Therapeutic Agents
ε-PL can be used to create drug delivery systems that provide controlled and sustained release of therapeutic agents. This is achieved through various mechanisms, including the formation of hydrogels, nanoparticles, and micelles that encapsulate the drug and release it in response to specific stimuli.
Hydrogel Formation: ε-PL can form hydrogels that encapsulate drugs and release them slowly over time. The gelation properties of ε-PL can be tuned to achieve desired release profiles.
Nanoparticle Encapsulation: ε-PL can be used to create nanoparticles that encapsulate drugs, protecting them from degradation and providing controlled release. These nanoparticles can be engineered to release the drug in response to pH changes, temperature shifts, or enzymatic activity.
Micelle Formation: ε-PL-based micelles can encapsulate hydrophobic drugs in their core, enhancing solubility and stability. The micelles can release the drug in response to environmental triggers, ensuring targeted and controlled delivery.
Targeted Drug Delivery
ε-PL can be functionalized with targeting ligands or molecules that enable the specific delivery of therapeutic agents to diseased tissues or cells. This targeted approach improves the efficacy of the treatment while minimizing off-target effects and toxicity.
Ligand Attachment: ε-PL can be conjugated with targeting ligands, such as antibodies, peptides, or small molecules, that bind specifically to receptors on the target cells.
Receptor-Mediated Targeting: Functionalized ε-PL-based delivery systems can selectively bind to receptors overexpressed on diseased cells, facilitating targeted delivery and enhancing therapeutic outcomes.
Applications in Biomedical Fields
Cancer Therapy
ε-PL-based drug delivery systems hold significant promise in cancer therapy. Their ability to enhance cellular uptake, provide controlled release, and target cancer cells makes them ideal for delivering chemotherapeutic agents and genetic material.
Chemotherapy: ε-PL nanoparticles and hydrogels can encapsulate chemotherapeutic drugs, providing sustained release and reducing systemic toxicity. Functionalization with targeting ligands ensures selective delivery to cancer cells.
Gene Therapy: ε-PL can form complexes with nucleic acids, protecting them from degradation and facilitating efficient gene delivery. These polyplexes can be designed to release the genetic material in response to specific intracellular conditions, improving gene expression and therapeutic outcomes.
Antimicrobial Therapy
ε-PL's broad-spectrum antimicrobial activity makes it valuable in treating infections and preventing biofilm formation on medical devices.
Antimicrobial Coatings: ε-PL can be incorporated into coatings for medical devices, such as catheters and implants, to prevent microbial colonization and biofilm formation. This reduces the risk of infections and improves patient outcomes.
Topical Formulations: ε-PL-based hydrogels and creams can be used to treat skin infections and wounds, providing sustained antimicrobial activity and promoting healing.
Tissue Engineering
In tissue engineering, ε-PL can be used to create scaffolds and hydrogels that support cell growth and tissue regeneration.
Scaffolds: ε-PL-based scaffolds provide a biocompatible and biodegradable matrix for cell attachment and growth. These scaffolds can be loaded with growth factors or drugs to enhance tissue regeneration.
Injectable Hydrogels: ε-PL hydrogels can be injected into damaged tissues, where they gel and provide a supportive environment for cell proliferation and differentiation. This approach is useful for repairing cartilage, bone, and soft tissues.
Challenges and Future Directions
Overcoming Production Costs
The production of ε-PL through bacterial fermentation can be costly. Efforts to optimize fermentation processes and scale up production are essential to reduce costs and make ε-PL-based applications more commercially viable.
Ensuring Long-Term Stability
While ε-PL enhances the stability of drug formulations, ensuring the long-term stability and shelf life of ε-PL-based delivery systems remains a challenge. Research into robust formulations and storage conditions is necessary to maintain efficacy over extended periods.
Addressing Potential Immunogenicity
Although ε-PL is generally biocompatible, repeated administration may pose a risk of immunogenicity. Comprehensive preclinical and clinical testing is required to assess and mitigate any immunogenic responses, ensuring the safety of ε-PL-based therapies.
Expanding Applications
Ongoing research into advanced formulations and novel applications of ε-PL is likely to expand its use in various biomedical fields. Innovations in drug delivery technology, tissue engineering, and antimicrobial therapy will enhance the versatility and impact of ε-PL.
Conclusion
ε-Polylysine hydrochloride is a versatile and promising material for enhancing drug delivery systems and various biomedical applications. Its antimicrobial properties, ability to enhance cellular uptake, and potential for controlled and targeted delivery make it an invaluable tool in modern medicine. Understanding the mechanisms of action of ε-PL provides a foundation for developing innovative therapies and improving existing treatments. As research continues and technological advancements are made, ε-PL is poised to play a significant role in the future of drug delivery and biomedical engineering.