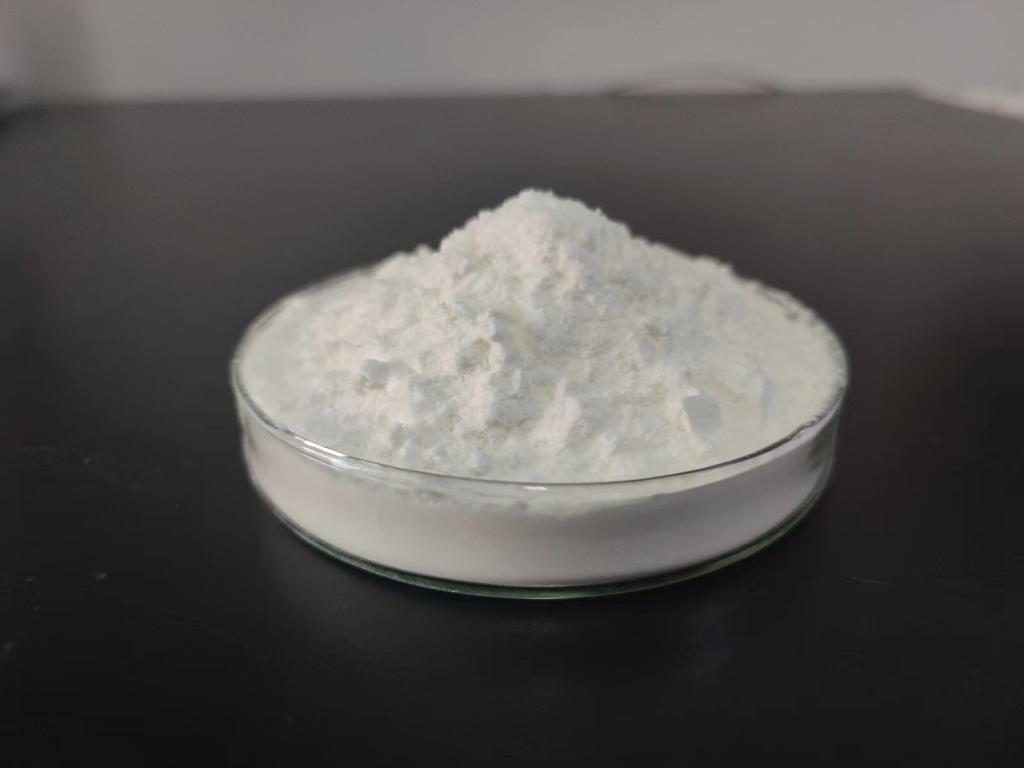
ε-Polylysine hydrochloride (ε-PL) has emerged as a promising natural antimicrobial agent with diverse applications in various industries, including food preservation, medical care, pharmaceuticals, agriculture, and environmental sustainability. However, the production of ε-PL presents several challenges that need to be addressed to ensure its scalability, cost-effectiveness, and sustainability. This article explores the challenges associated with ε-PL production and proposes strategies to overcome them, paving the way for wider adoption and utilization of this valuable biotechnological tool.
Understanding the Production Process of ε-Polylysine Hydrochloride
Before delving into the challenges, it's essential to understand the production process of ε-PL. ε-PL is typically produced through microbial fermentation using certain strains of Streptomyces bacteria. The fermentation process involves cultivating the bacteria in a suitable medium, usually containing glucose or other carbohydrates as carbon sources, nitrogen sources, minerals, and growth factors. During fermentation, the bacteria produce ε-PL, which can then be extracted and purified for various applications.
Challenges in ε-PL Production
Low Yield and Productivity
Suboptimal Fermentation Conditions: The yield of ε-PL can be affected by various factors such as pH, temperature, agitation, aeration, and nutrient availability. Suboptimal fermentation conditions can lead to low productivity and inconsistent product quality.
Strain Selection: Not all strains of Streptomyces bacteria produce ε-PL efficiently. Selecting high-yielding strains with robust production capabilities is crucial for maximizing productivity.
Cost of Production
Raw Material Costs: The cost of raw materials, including carbon and nitrogen sources, minerals, and growth factors, contributes significantly to the overall production cost of ε-PL. Finding cost-effective sources of these materials is essential for reducing production costs.
Energy Consumption: The energy required for fermentation, agitation, sterilization, and downstream processing adds to the production cost. Implementing energy-efficient practices and optimizing process parameters can help minimize energy consumption.
Downstream Processing
Purification and Recovery: The purification of ε-PL from fermentation broth involves multiple steps, including filtration, centrifugation, chromatography, and drying. These steps can be complex, time-consuming, and expensive, leading to high production costs.
Product Losses: Losses during purification and recovery processes reduce overall yield and increase production costs. Improving recovery efficiency and minimizing product losses are critical for enhancing process economics.
Scale-Up Challenges
Maintaining Productivity: Scaling up ε-PL production from laboratory-scale to industrial-scale presents challenges in maintaining productivity and product quality. Factors such as mixing, oxygen transfer, heat transfer, and mass transfer become more critical at larger scales.
Process Control: Ensuring uniformity and consistency in large-scale fermentation processes requires robust process control systems and monitoring techniques.
Regulatory Compliance
Quality and Safety Standards: Meeting regulatory requirements for product quality, safety, and purity is essential for commercializing ε-PL. Compliance with Good Manufacturing Practices (GMP) and other regulatory standards adds complexity and cost to production processes.
Strategies to Overcome Production Challenges
Optimization of Fermentation Conditions
Process Parameter Optimization: Conducting systematic studies to optimize fermentation conditions, including pH, temperature, agitation, aeration, and nutrient concentrations, to maximize ε-PL yield and productivity.
Strain Improvement: Employing strain improvement techniques such as mutagenesis, strain selection, and genetic engineering to develop high-yielding Streptomyces strains with enhanced ε-PL production capabilities.
Utilization of Renewable Resources
Carbon Source Optimization: Exploring alternative and renewable carbon sources, such as agricultural residues, lignocellulosic biomass, and waste streams, to reduce reliance on expensive sugars and lower production costs.
Nitrogen Source Selection: Identifying cost-effective nitrogen sources, such as agricultural by-products, organic waste, and microbial by-products, to replace conventional nitrogen sources and enhance process sustainability.
Process Intensification
Bioreactor Design: Designing and optimizing bioreactor systems for efficient ε-PL production, including bioreactor geometry, mixing strategies, oxygen transfer rates, and fermentation kinetics, to maximize productivity and minimize energy consumption.
High-Cell-Density Fermentation: Implementing high-cell-density fermentation techniques, such as fed-batch and continuous fermentation, to achieve higher ε-PL titers and improve process economics.
Downstream Process Optimization
Integrated Purification Processes: Developing integrated and streamlined purification processes that combine multiple steps, such as precipitation, extraction, chromatography, and filtration, to reduce processing time, cost, and product losses.
Advanced Separation Technologies: Utilizing advanced separation technologies, such as membrane filtration, adsorption, and crystallization, to improve purification efficiency, product purity, and recovery yields.
Scale-Up Strategies
Pilot-Scale Testing: Conducting pilot-scale trials to validate fermentation and downstream processing protocols, optimize process parameters, and identify potential scale-up challenges before transitioning to full-scale production.
Modular Production Systems: Implementing modular production systems that allow for flexible and scalable operation, enabling rapid scale-up and customization according to specific production requirements.
Technological Innovations
Automation and Control Systems: Incorporating advanced automation and control systems into fermentation and downstream processing operations to enhance process monitoring, optimization, and reproducibility, while reducing labor costs and human errors.
Data Analytics and Process Modeling: Utilizing data analytics tools and process modeling techniques to analyze large datasets, identify process trends, optimize process parameters, and predict process outcomes, leading to improved process efficiency and productivity.
Collaborative Partnerships and Knowledge Sharing
Industry-Academia Collaboration: Fostering collaborative partnerships between industry and academia to exchange knowledge, share best practices, and co-develop innovative solutions for ε-PL production challenges.
Technology Transfer: Facilitating technology transfer and knowledge dissemination through conferences, workshops, training programs, and online platforms to accelerate the adoption of advanced production technologies and practices.
Conclusion
The production of ε-Polylysine hydrochloride presents several challenges, including low yield and productivity, high production costs, downstream processing complexities, scale-up challenges, and regulatory compliance requirements. However, with strategic interventions and innovative approaches, these challenges can be overcome, paving the way for scalable, cost-effective, and sustainable production of ε-PL. Optimization of fermentation conditions, utilization of renewable resources, process intensification, downstream process optimization, scale-up strategies, technological innovations, and collaborative partnerships are key strategies to address production challenges and unlock the full potential of ε-PL as a valuable biotechnological tool. By overcoming these challenges, ε-PL production can be streamlined, leading to wider adoption and utilization in various industries, including food and beverage, healthcare, agriculture, and environmental sustainability, ultimately benefiting society and the economy.