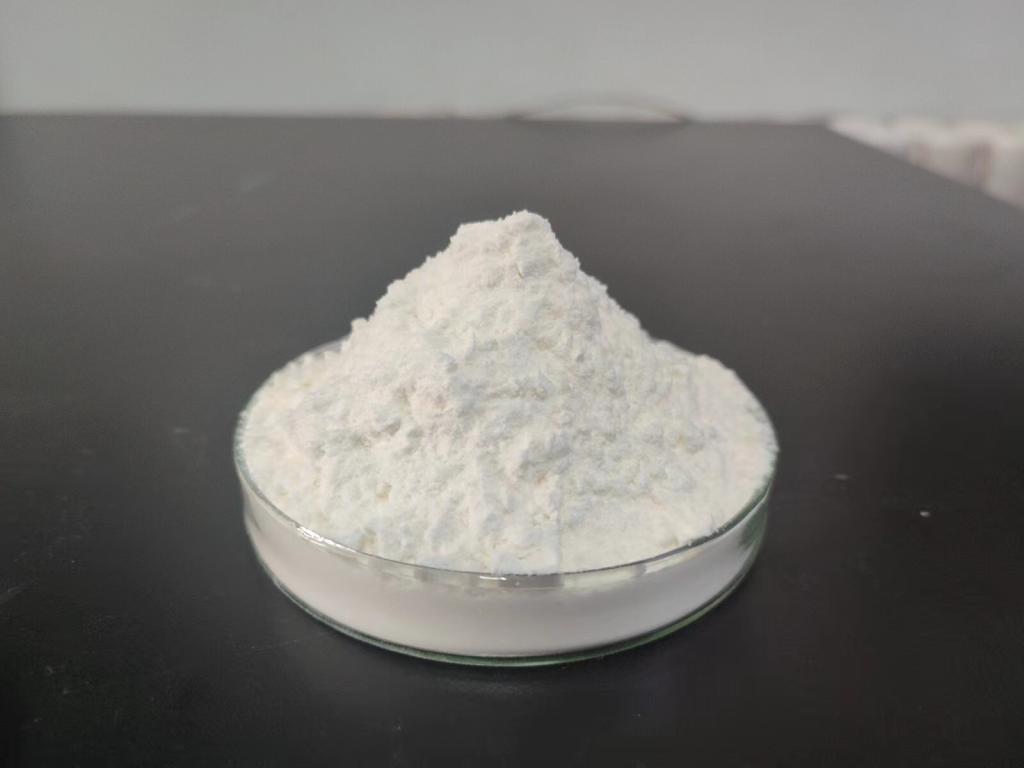
ε-Polylysine hydrochloride, a naturally occurring antimicrobial peptide, has gained prominence for its applications in the food industry, pharmaceuticals, and various other fields. Ongoing research endeavors are focused on optimizing production processes to increase efficiency and cost-effectiveness, addressing key challenges in scaling up ε-polylysine hydrochloride manufacturing. This article provides an in-depth exploration of the current state of research, highlighting strategies employed to enhance production, overcome challenges, and unlock the full potential of this versatile compound.
1. Introduction:
ε-Polylysine hydrochloride, derived from microbial fermentation, has demonstrated significant antimicrobial properties and has found utility in diverse industries. The demand for this compound has spurred ongoing research aimed at refining production processes, with the ultimate goals of improving efficiency and cost-effectiveness. This article delves into the multifaceted aspects of ε-polylysine hydrochloride production, examining current challenges and innovative solutions in the quest for optimization.
2. ε-Polylysine Hydrochloride: Properties and Applications:
Before delving into production processes, understanding the properties and applications of ε-polylysine hydrochloride is crucial. This naturally occurring cationic peptide exhibits antimicrobial activity against a broad spectrum of microorganisms and has been utilized as a preservative in food products, a coating for medical devices, and a potential therapeutic agent. A thorough comprehension of its properties sets the stage for informed optimization strategies.
3. Microbial Fermentation: The Foundation of Production:
Microbial fermentation stands as the cornerstone of ε-polylysine hydrochloride production. The choice of microbial strains, fermentation conditions, and cultivation methods profoundly influences the yield and purity of the final product. Ongoing research is scrutinizing these variables, aiming to identify optimal conditions that maximize ε-polylysine hydrochloride production while ensuring consistency and quality.
4. Strain Improvement and Genetic Engineering:
Strain improvement through traditional methods and modern genetic engineering techniques plays a pivotal role in enhancing ε-polylysine hydrochloride production. Researchers are exploring the genetic manipulation of microbial strains to improve their productivity, tolerance to environmental factors, and overall efficiency in producing ε-polylysine hydrochloride.
5. Bioprocess Engineering: Scaling Up for Commercial Production:
Transitioning from laboratory-scale production to commercial-scale manufacturing poses inherent challenges. Bioprocess engineering encompasses the optimization of fermentation conditions, downstream processing, and purification methods to achieve high yields with reduced production costs. Advances in bioprocess engineering are critical for meeting the increasing demand for ε-polylysine hydrochloride on an industrial scale.
6. Downstream Processing and Purification Strategies:
Efficient downstream processing is imperative to isolate and purify ε-polylysine hydrochloride from the fermentation broth. Ongoing research explores novel separation and purification techniques, such as chromatography, membrane filtration, and crystallization, with the aim of streamlining processes, reducing energy consumption, and improving overall yield.
7. Quality Control and Characterization:
Ensuring the consistent quality of ε-polylysine hydrochloride is paramount for its successful integration into various applications. Researchers are developing robust quality control measures, including analytical techniques for the characterization of ε-polylysine hydrochloride, to meet regulatory standards and guarantee the reliability of the final product.
8. Challenges in Production Optimization:
Despite the progress made, challenges in ε-polylysine hydrochloride production persist. These include the need for cost-effective substrates, optimizing fermentation conditions for different microbial strains, and addressing environmental concerns associated with large-scale production. Tackling these challenges requires a multidisciplinary approach and collaboration among researchers, industry stakeholders, and regulatory bodies.
9. Sustainable Production Practices:
As the focus on sustainability grows, researchers are exploring environmentally friendly and sustainable production practices for ε-polylysine hydrochloride. This includes the utilization of renewable resources, waste valorization, and the development of eco-friendly processes to minimize the ecological footprint of ε-polylysine hydrochloride production.
10. Future Perspectives and Commercialization:
The optimization of ε-polylysine hydrochloride production processes sets the stage for its broader commercialization and integration into diverse industries. Future perspectives include the development of cost-effective and sustainable production platforms, exploration of novel applications, and continued collaboration between academia and industry to drive innovation.
11. Conclusion:
The ongoing research dedicated to optimizing ε-polylysine hydrochloride production processes reflects the dynamic intersection of science, technology, and industry. As researchers continue to unravel the complexities of microbial fermentation, genetic engineering, and bioprocess engineering, the potential for ε-polylysine hydrochloride to play a pivotal role in various applications becomes increasingly apparent. This journey towards optimization underscores the collective commitment to advancing scientific knowledge and meeting the demands of a rapidly evolving global market.