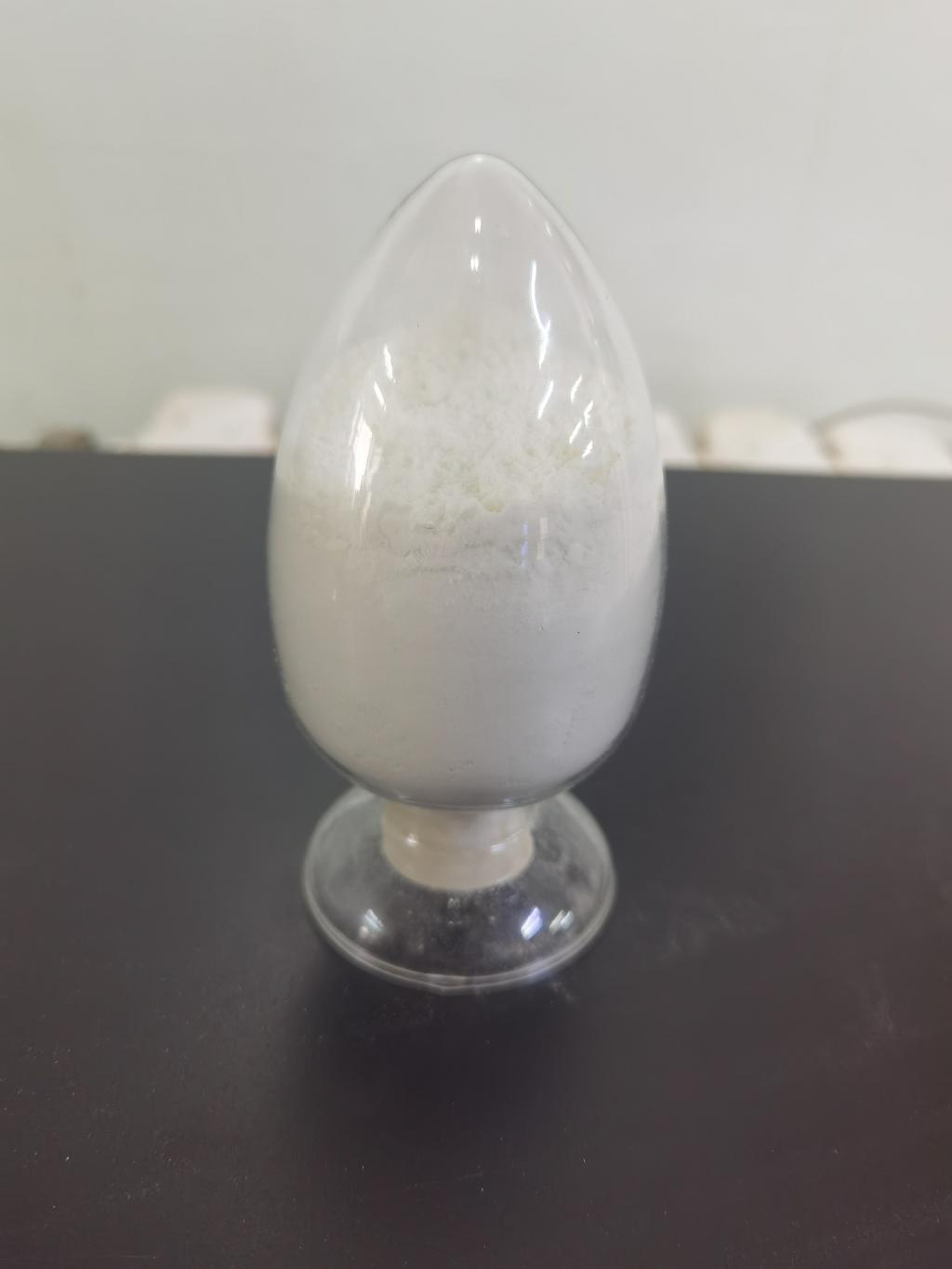
The field of targeted therapy aims to deliver therapeutic agents directly to diseased cells or tissues, minimizing side effects and enhancing treatment efficacy. ε-Polylysine hydrochloride (ε-PLH), a naturally occurring cationic polymer, has garnered attention for its antimicrobial properties, biocompatibility, and biodegradability. Its potential in targeted therapies is being explored through novel delivery systems that can improve its therapeutic index and ensure precise delivery to target sites. This article delves into the novel delivery systems for ε-PLH in targeted therapies, examining their mechanisms, benefits, and potential applications.
Understanding ε-Polylysine Hydrochloride
Chemical Structure and Properties
ε-Polylysine hydrochloride is a homopolymer of L-lysine, produced by the fermentation of Streptomyces albulus. Its structure consists of lysine residues linked by ε-amino bonds, imparting a strong cationic charge. This positive charge facilitates interaction with negatively charged molecules and cell membranes, enhancing its potential as a therapeutic agent.
Therapeutic Potential
The therapeutic potential of ε-PLH lies in its antimicrobial activity, ability to disrupt biofilms, and potential to interact with cell membranes. These properties make it suitable for a range of medical applications, including as an antimicrobial agent, a drug delivery vehicle, and a component in tissue engineering.
Novel Delivery Systems
Nanoparticles
Polymeric Nanoparticles
Polymeric nanoparticles (NPs) made from biodegradable polymers such as polylactic acid (PLA), polyglycolic acid (PGA), and their copolymers (PLGA) are ideal carriers for ε-PLH. These nanoparticles can encapsulate ε-PLH, protecting it from degradation and ensuring controlled release at the target site. The surface of these NPs can be functionalized with targeting ligands to enhance specificity towards diseased cells.
Lipid-Based Nanoparticles
Lipid-based nanoparticles, including liposomes and solid lipid nanoparticles (SLNs), offer another promising delivery system for ε-PLH. These carriers can encapsulate both hydrophilic and hydrophobic agents, providing versatility in drug formulation. Liposomes can fuse with cell membranes, facilitating the direct delivery of ε-PLH into the cytoplasm, while SLNs offer a stable matrix for sustained release.
Hydrogels
Hydrogels are three-dimensional networks of hydrophilic polymers capable of holding large amounts of water. ε-PLH can be incorporated into hydrogels to create injectable or implantable delivery systems. These hydrogels can provide localized and sustained release of ε-PLH, enhancing its therapeutic effect while minimizing systemic exposure.
Micelles
Micelles are self-assembling colloidal structures formed by amphiphilic molecules. ε-PLH can be incorporated into micelles to improve its solubility and stability in aqueous environments. The core of the micelle can encapsulate ε-PLH, while the hydrophilic shell ensures biocompatibility and prolonged circulation time in the bloodstream.
Dendrimers
Dendrimers are highly branched, tree-like polymers with a high degree of surface functionality. They can encapsulate ε-PLH within their interior cavities or conjugate ε-PLH to their surface. Dendrimers offer precise control over size, shape, and surface chemistry, making them suitable for targeted delivery applications. Their multivalent surface can be functionalized with targeting ligands and imaging agents, enabling targeted therapy and diagnostic applications.
Targeted Delivery Systems
Ligand-Targeted Delivery
Targeting ligands such as antibodies, peptides, or small molecules can be conjugated to ε-PLH or its delivery carriers to enhance specificity. These ligands bind to receptors overexpressed on target cells, facilitating the selective delivery of ε-PLH to diseased tissues. For example, folate receptors are overexpressed in certain cancer cells, and folate-conjugated ε-PLH can be used to target these cells specifically.
Stimuli-Responsive Delivery
Stimuli-responsive delivery systems release their payload in response to specific triggers such as pH, temperature, or enzymatic activity. ε-PLH can be incorporated into these systems to achieve site-specific release. For instance, a pH-sensitive delivery system can release ε-PLH in the acidic environment of a tumor, ensuring high local concentrations at the target site.
Mechanisms of Action in Targeted Therapies
Antimicrobial Activity
ε-PLH's antimicrobial properties are well-documented. In targeted therapies, ε-PLH can be delivered directly to sites of infection or biofilm formation. Its ability to disrupt bacterial cell membranes and inhibit biofilm growth makes it a powerful tool in treating chronic infections and preventing implant-related infections.
Biofilm Disruption
Biofilms are protective matrices produced by bacterial colonies that render them resistant to antibiotics. ε-PLH can penetrate and disrupt these biofilms, enhancing the efficacy of conventional antibiotics. Targeted delivery of ε-PLH to biofilm sites can significantly improve treatment outcomes for biofilm-associated infections.
Cell Membrane Interaction
ε-PLH interacts with cell membranes due to its cationic nature. In targeted therapies, ε-PLH can be directed towards cancer cells, which often exhibit negatively charged membranes. This interaction can lead to the disruption of cancer cell membranes, inducing cell death and inhibiting tumor growth.
Immunomodulation
Emerging research suggests that ε-PLH may have immunomodulatory effects, enhancing the body's immune response to infections and tumors. By delivering ε-PLH directly to immune cells or tumor sites, it may be possible to boost the immune response and improve therapeutic outcomes.
Applications in Targeted Therapies
Cancer Therapy
Direct Anticancer Effects
ε-PLH can be used in cancer therapy due to its ability to disrupt cell membranes. Targeted delivery of ε-PLH to tumor cells can induce apoptosis and inhibit tumor growth. Additionally, ε-PLH can be used to deliver chemotherapeutic agents directly to cancer cells, reducing systemic toxicity and improving efficacy.
Combination Therapy
Combining ε-PLH with other therapeutic agents can enhance treatment outcomes. For example, ε-PLH can be used to disrupt the tumor microenvironment, making cancer cells more susceptible to chemotherapy and radiation therapy. This combination approach can overcome resistance mechanisms and improve patient prognosis.
Antimicrobial Therapy
Treatment of Chronic Infections
Chronic infections often involve biofilms that are resistant to conventional antibiotics. ε-PLH can disrupt these biofilms and enhance the penetration of antibiotics, improving treatment outcomes. Targeted delivery of ε-PLH to infection sites can reduce the required dosage of antibiotics and minimize side effects.
Prevention of Implant-Related Infections
Medical implants are prone to bacterial colonization and biofilm formation, leading to infections. Coating implants with ε-PLH or using ε-PLH-loaded delivery systems can prevent microbial colonization and biofilm formation, reducing the risk of implant-related infections.
Wound Healing
Antimicrobial Wound Dressings
ε-PLH can be incorporated into wound dressings to provide antimicrobial protection and promote healing. These dressings can prevent infection at the wound site and create a conducive environment for tissue regeneration.
Controlled Release Systems
Controlled release systems can deliver ε-PLH to wounds over an extended period, ensuring sustained antimicrobial activity. This approach can reduce the frequency of dressing changes and improve patient comfort and compliance.
Challenges and Future Directions
Stability and Bioavailability
One of the primary challenges in developing ε-PLH-based delivery systems is ensuring the stability and bioavailability of ε-PLH. Researchers are exploring various strategies to improve the stability of ε-PLH in biological environments and enhance its bioavailability at target sites.
Safety and Toxicity
While ε-PLH is generally considered safe, its toxicity profile needs to be thoroughly evaluated, especially in the context of long-term use and high-dose applications. Understanding the potential side effects and developing strategies to mitigate them is crucial for the successful translation of ε-PLH-based therapies.
Regulatory Approval
Achieving regulatory approval for ε-PLH-based delivery systems involves rigorous testing and validation. Ensuring that these systems meet safety, efficacy, and quality standards is essential for their clinical application. Collaboration between researchers, industry, and regulatory bodies will be vital in this process.
Personalized Medicine
The development of personalized ε-PLH-based therapies tailored to individual patient needs is a promising direction. This approach involves designing delivery systems that account for patient-specific factors such as genetic profile, disease stage, and treatment response, optimizing therapeutic outcomes.
Conclusion
ε-Polylysine hydrochloride holds significant promise in targeted therapies due to its antimicrobial properties, biocompatibility, and ability to interact with cell membranes. Novel delivery systems, including nanoparticles, hydrogels, micelles, and dendrimers, are enhancing the therapeutic potential of ε-PLH by ensuring precise and controlled delivery to target sites. These advancements open new avenues for the treatment of cancer, chronic infections, wound healing, and more. As research progresses and challenges are addressed, ε-PLH-based delivery systems are poised to play a transformative role in modern medicine, offering more effective and targeted therapeutic options for a range of diseases.