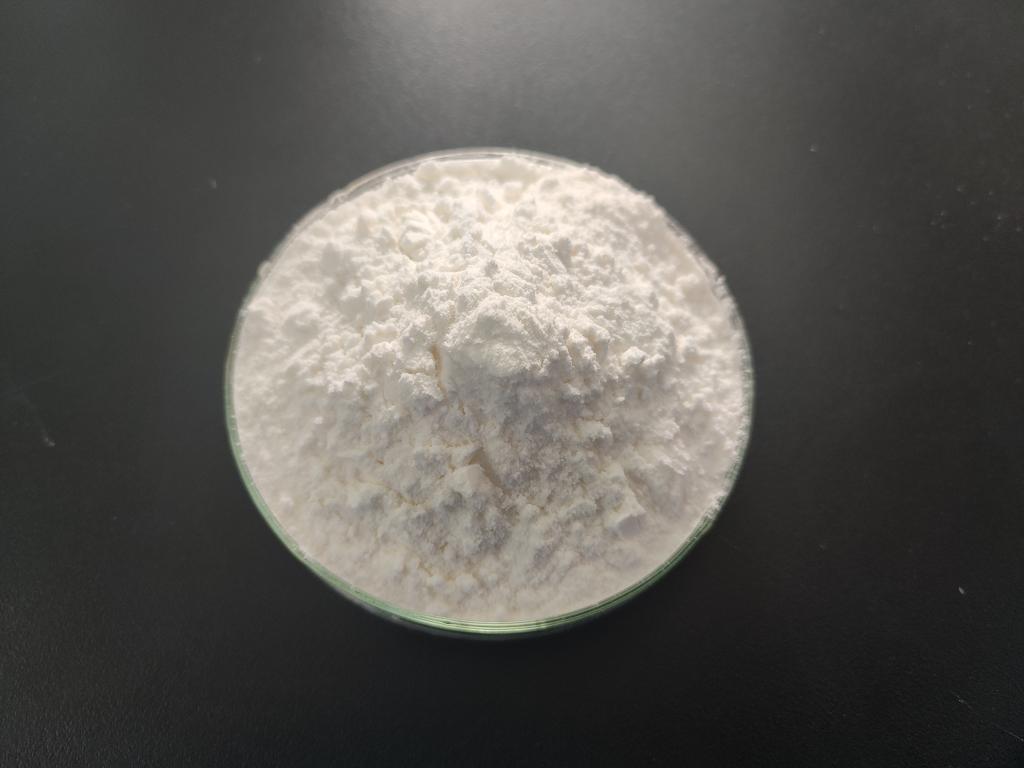
ε-Polylysine hydrochloride (ε-PL) is a naturally occurring polycationic peptide produced by certain strains of Streptomyces albulus. Known for its potent antimicrobial properties, ε-PL has been widely used in food preservation and has potential applications in various fields, including medicine and environmental protection. Understanding the biodegradation pathways of ε-PL is essential for assessing its environmental impact and optimizing its use in various applications. This article delves into the biodegradation mechanisms of ε-PL, exploring the factors influencing its degradation, the microbial and enzymatic pathways involved, and the implications for sustainable use.
Structure and Properties of ε-Polylysine Hydrochloride
ε-Polylysine hydrochloride is composed of multiple lysine residues linked by peptide bonds through the ε-amino groups. This unique structure endows ε-PL with its polycationic nature, contributing to its antimicrobial efficacy by interacting with microbial cell membranes. The biodegradability of ε-PL is a key factor in its environmental footprint, influencing its persistence and impact in various ecosystems.
Factors Influencing Biodegradation
The biodegradation of ε-PL is influenced by several factors, including environmental conditions, microbial community composition, and the presence of specific enzymes. Key factors include:
pH and Temperature: ε-PL degradation can be affected by pH and temperature, with optimal conditions promoting faster degradation. Acidic or alkaline conditions, along with varying temperatures, can impact enzyme activity and microbial growth.
Microbial Community: The diversity and abundance of microbial communities play a crucial role in ε-PL degradation. Certain bacteria and fungi possess the enzymatic machinery required to break down ε-PL.
Enzymatic Activity: Specific enzymes, such as proteases and peptidases, are essential for the hydrolysis of ε-PL into smaller peptides and amino acids, facilitating further microbial degradation.
Microbial and Enzymatic Pathways
Biodegradation of ε-PL involves both microbial and enzymatic pathways, which work synergistically to break down the polymer into its constituent components. The primary steps in the biodegradation process include:
Initial Hydrolysis:
The initial step in ε-PL degradation involves the hydrolysis of peptide bonds by extracellular enzymes, such as proteases and peptidases. These enzymes cleave the ε-polylysine into smaller peptides and individual lysine residues.
Microbial Uptake and Metabolism:
The smaller peptides and lysine residues produced from the initial hydrolysis are taken up by microbial cells. Inside the cells, these compounds undergo further degradation through metabolic pathways. Lysine, for instance, can be catabolized through the lysine degradation pathway, leading to the production of acetyl-CoA and other metabolites that enter central metabolic pathways such as the tricarboxylic acid (TCA) cycle.
Mineralization:
The final step in the biodegradation process is the mineralization of the metabolites into carbon dioxide, water, and other inorganic compounds. This step is facilitated by a diverse range of microorganisms capable of utilizing the metabolic intermediates produced from ε-PL degradation.
Key Microorganisms Involved
Several microorganisms have been identified as key players in the biodegradation of ε-PL. These include:
Bacteria: Certain bacterial strains, such as Bacillus and Pseudomonas species, possess the necessary enzymes for ε-PL degradation. These bacteria can utilize ε-PL as a nitrogen and carbon source, facilitating its breakdown and mineralization.
Fungi: Fungal species, including Aspergillus and Penicillium, have also been shown to degrade ε-PL. Fungi produce a wide range of extracellular enzymes capable of hydrolyzing complex polymers, making them effective in ε-PL biodegradation.
Environmental Implications
The biodegradability of ε-PL has significant implications for its environmental impact and sustainability. Key considerations include:
Environmental Persistence: The rate of ε-PL degradation determines its persistence in the environment. Rapid degradation reduces the risk of accumulation and potential adverse effects on ecosystems.
Ecotoxicity: The breakdown products of ε-PL, primarily lysine and smaller peptides, are generally considered non-toxic. However, comprehensive ecotoxicity assessments are necessary to evaluate the impact of ε-PL and its degradation products on various environmental compartments.
Regulatory Considerations: Understanding the biodegradation pathways of ε-PL is crucial for regulatory approval and compliance. Regulatory agencies require detailed information on the environmental fate and impact of substances to ensure their safe use.
Applications and Future Directions
Exploring the biodegradation pathways of ε-PL opens up opportunities for optimizing its use in various applications. Potential areas of focus include:
Food Preservation: Enhancing the stability and controlled degradation of ε-PL in food systems to extend shelf life while ensuring safety and quality.
Biomedical Applications: Developing ε-PL-based antimicrobial coatings and materials with predictable degradation profiles for use in medical devices and implants.
Environmental Protection: Leveraging the biodegradability of ε-PL for environmentally friendly pest control and agricultural applications, minimizing environmental impact.
Conclusion
Understanding the biodegradation pathways of ε-Polylysine hydrochloride is essential for optimizing its use and assessing its environmental impact. By elucidating the factors influencing its degradation, the microbial and enzymatic pathways involved, and the environmental implications, we can harness the potential of ε-PL in a sustainable and responsible manner. Continued research and innovation in this field will contribute to the development of effective and eco-friendly applications, promoting public health and environmental protection.