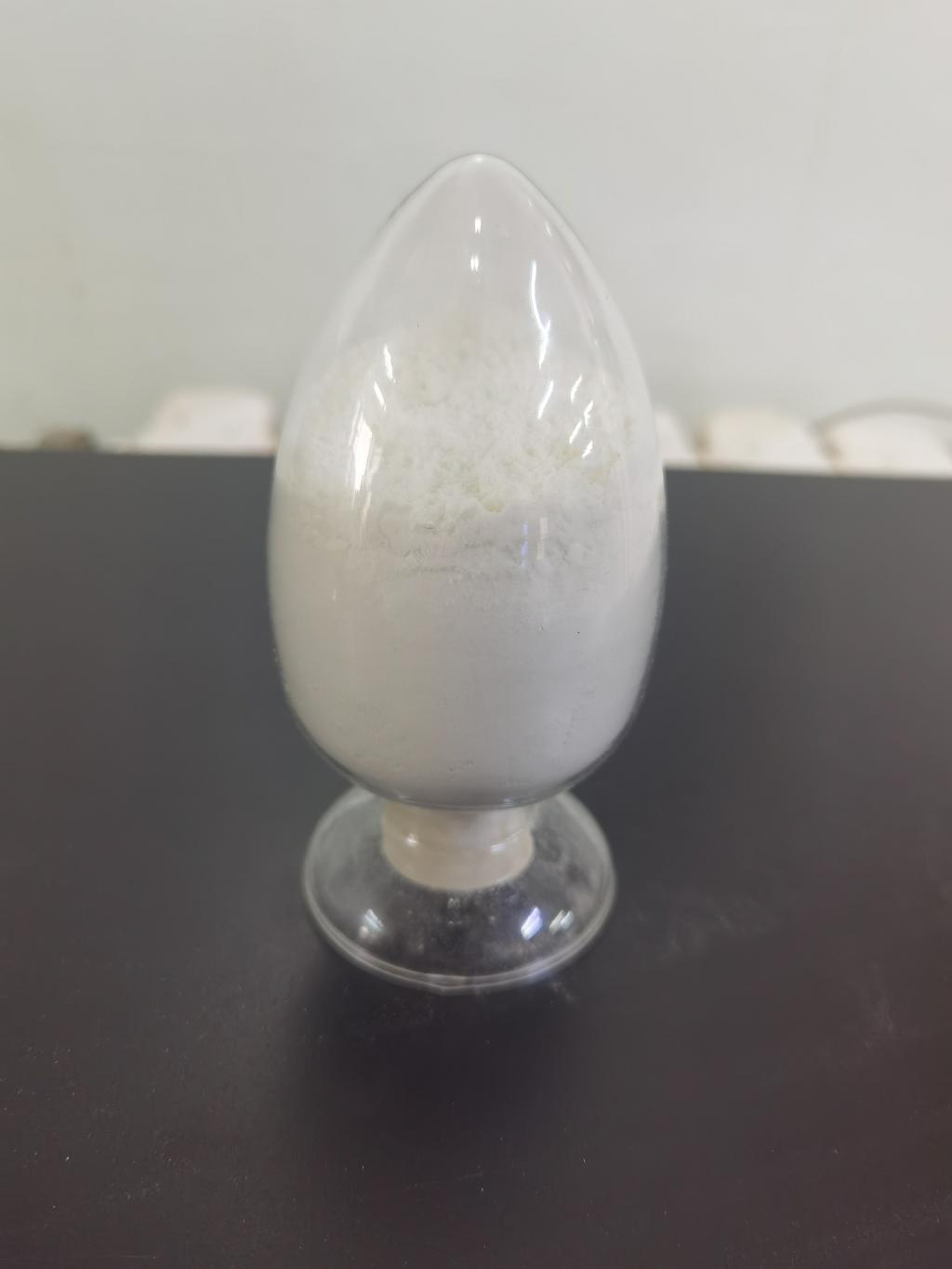
ε-Polylysine hydrochloride, an antimicrobial peptide with a natural origin, has gained significant attention for its potent antibacterial properties. This article delves into the intricate mechanisms underlying the antibacterial prowess of ε-polylysine hydrochloride. From its unique structure to the disruption of microbial cell membranes and the inhibition of biofilm formation, this exploration aims to decode the multifaceted mechanisms that make ε-polylysine hydrochloride a formidable antibacterial powerhouse.
Introduction:
As bacterial resistance to traditional antibiotics poses an escalating global health threat, the exploration of alternative antibacterial agents becomes imperative. ε-Polylysine hydrochloride, a naturally occurring antimicrobial peptide, has emerged as a promising antibacterial powerhouse. This article aims to unravel the complex mechanisms that contribute to the antibacterial efficacy of ε-polylysine hydrochloride, shedding light on its potential applications and implications in the fight against bacterial infections.
Origins and Production of ε-Polylysine Hydrochloride:
Biosynthesis in Bacteria:
ε-Polylysine is synthesized by certain strains of bacteria, particularly through a biosynthetic pathway that involves the polymerization of lysine residues. This natural origin contributes to its safety profile and aligns with the growing preference for naturally derived antimicrobial agents.
Fermentation and Extraction:
Commercial production of ε-polylysine hydrochloride involves fermentation processes using specific bacterial strains. Subsequent extraction and purification processes yield the hydrochloride form, which is utilized for various applications due to its stability and solubility.
Unique Structural Features:
Polymeric Nature:
ε-Polylysine is characterized by its polymeric structure, featuring multiple lysine residues linked together. This unique structure imparts distinct properties to ε-polylysine hydrochloride, contributing to its antimicrobial efficacy.
Positive Charge Distribution:
The polymeric structure of ε-polylysine hydrochloride results in an overall positive charge. This positive charge is a key factor in its interactions with microbial cell membranes, facilitating its antibacterial effects.
Mechanisms of Antibacterial Action:
Disruption of Microbial Cell Membranes:
The primary mechanism underlying the antibacterial activity of ε-polylysine hydrochloride involves the disruption of microbial cell membranes. Its positively charged structure allows it to interact with the negatively charged components of bacterial cell walls, leading to membrane destabilization.
Formation of Pores:
ε-Polylysine hydrochloride's interaction with microbial cell membranes induces the formation of pores. These pores compromise the integrity of the membrane, leading to leakage of cellular contents and eventual cell death. The formation of pores is a crucial step in its bactericidal action.
Selectivity and Spectrum of Activity:
While ε-polylysine hydrochloride exhibits broad-spectrum antibacterial activity, its selectivity for certain bacterial species enhances its effectiveness. Variations in the composition of bacterial cell walls contribute to this selectivity, making ε-polylysine hydrochloride a targeted antibacterial agent.
Inhibition of Biofilm Formation:
Biofilm as a Protective Barrier:
Bacterial biofilms, protective layers formed by microorganisms, pose a significant challenge in combating infections. Biofilms contribute to bacterial resistance and persistence, making traditional treatments less effective.
Biofilm-Inhibiting Properties:
ε-Polylysine hydrochloride has demonstrated the ability to inhibit the formation of bacterial biofilms. By disrupting the initial stages of biofilm development, ε-polylysine hydrochloride addresses a critical aspect of bacterial survival strategies, enhancing its antibacterial effectiveness.
Potential Applications in Medical Settings:
Wound Care and Infection Prevention:
The antibacterial properties of ε-polylysine hydrochloride make it a potential candidate for wound care formulations. Its ability to inhibit biofilm formation could play a crucial role in preventing infections in wounds and promoting effective wound healing.
Implant and Medical Device Coatings:
Bacterial colonization on medical implants and devices poses significant risks. Coating these surfaces with ε-polylysine hydrochloride could serve as a preventive measure, reducing the likelihood of bacterial infections associated with implanted medical devices.
Challenges and Considerations:
Resistance Development:
Like any antimicrobial agent, the potential for bacterial resistance to ε-polylysine hydrochloride exists. Ongoing research is essential to monitor and understand resistance mechanisms, allowing for the development of strategies to mitigate this challenge.
Optimizing Formulations:
The effectiveness of ε-polylysine hydrochloride is influenced by various factors, including formulation conditions and the presence of other compounds. Optimizing formulations for specific applications is crucial to ensure its stability and antibacterial activity.
Future Directions and Implications:
Combination Therapies:
The potential use of ε-polylysine hydrochloride in combination therapies with traditional antibiotics could enhance their effectiveness and potentially reduce the risk of resistance development.
Biomedical Engineering Innovations:
Advances in biomedical engineering may lead to the development of innovative materials and devices incorporating ε-polylysine hydrochloride. This could open new avenues for antibacterial applications in medical settings.
Conclusion:
The antibacterial powerhouse that is ε-polylysine hydrochloride holds promise in revolutionizing our approach to combating bacterial infections. From its unique structural features to the disruption of microbial cell membranes and inhibition of biofilm formation, the multifaceted mechanisms underlying its antibacterial action make it a compelling candidate for various applications. As research progresses, ε-polylysine hydrochloride could emerge as a cornerstone in the development of next-generation antibacterial strategies, offering new hope in the face of bacterial resistance and challenging infections.