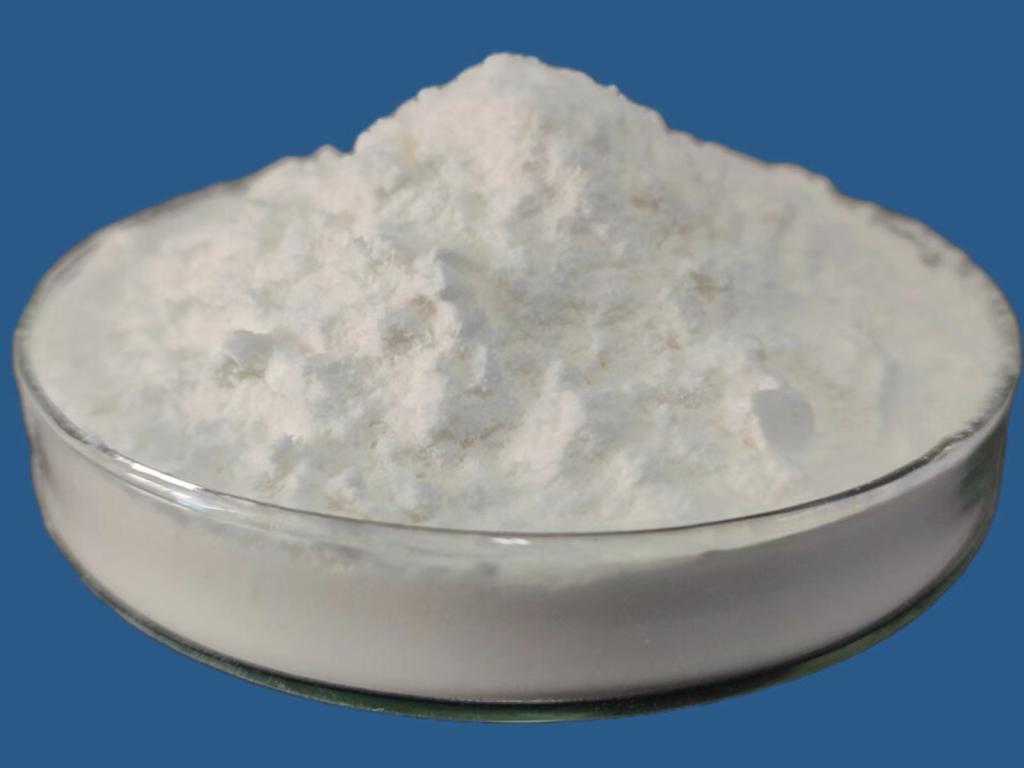
ε-Polylysine hydrochloride (ε-PL) is a biopolymer with significant potential due to its antimicrobial properties, biocompatibility, and versatility. Derived from bacterial fermentation, ε-PL has found applications across various fields, including food preservation, pharmaceuticals, and cosmetics. Recent advancements in its synthesis and modification are expanding its utility and improving its performance in different applications. This article explores the latest advancements in the synthesis and modification of ε-Polylysine hydrochloride, focusing on novel synthesis methods, structural modifications, and emerging applications.
Synthesis of ε-Polylysine Hydrochloride
Traditional Fermentation-Based Synthesis
Historically, ε-Polylysine hydrochloride has been produced through microbial fermentation. The process involves culturing specific bacterial strains, such as Streptomyces albulus, which are capable of producing ε-PL. This method remains widely used due to its efficiency and the natural origin of the product. Key steps include:
Inoculum Preparation: Cultivating a starter culture of Streptomyces albulus in a suitable medium.
Fermentation: Growing the inoculum in large-scale fermenters under controlled conditions (e.g., temperature, pH) to optimize ε-PL production.
Harvesting: Extracting ε-PL from the fermentation broth through processes like centrifugation and filtration.
Purification: Refining the extracted ε-PL using methods such as ion exchange chromatography or precipitation.
Despite its widespread use, traditional fermentation has limitations in terms of yield, cost, and scalability.
Advances in Fermentation Technology
Strain Improvement
Recent advancements focus on enhancing ε-PL yield through the development of genetically modified strains. Techniques such as genetic engineering, directed evolution, and synthetic biology are used to improve the productivity of Streptomyces strains. Examples include:
Genetic Engineering: Introducing or modifying genes involved in ε-PL biosynthesis to increase production levels.
Directed Evolution: Creating mutant strains through random mutagenesis and selecting those with improved ε-PL production capabilities.
Optimization of Fermentation Conditions
Innovations in fermentation technology have led to better control and optimization of production conditions:
Continuous Fermentation: Employing continuous fermentation processes can enhance productivity and reduce production costs by maintaining optimal conditions throughout the growth cycle.
Advanced Bioreactor Designs: Implementing new bioreactor technologies, such as airlift reactors or perfusion systems, can improve oxygen transfer and nutrient utilization, leading to higher yields.
Chemical Synthesis Methods
In addition to microbial fermentation, chemical synthesis methods have been explored for ε-PL production. These methods involve synthesizing ε-PL through chemical reactions rather than biological processes. Approaches include:
Solid-Phase Synthesis: Utilizing solid-phase peptide synthesis (SPPS) techniques to assemble ε-PL chains in a controlled manner.
Solution-Phase Synthesis: Employing solution-phase methods to create ε-PL with precise control over chain length and composition.
While chemical synthesis offers control over molecular structure, it often involves complex and costly processes compared to fermentation.
Modification of ε-Polylysine Hydrochloride
Structural Modifications
Modifying the structure of ε-PL can enhance its properties and expand its applications. Key areas of modification include:
Substitution and Functionalization
Side Chain Modifications: Introducing functional groups into the side chains of ε-PL can alter its interactions with biological systems and improve its antimicrobial efficacy. For example, adding hydrophobic groups can enhance its activity against certain pathogens.
Conjugation with Other Molecules: ε-PL can be conjugated with other bioactive molecules, such as drugs or targeting agents, to create hybrid compounds with improved therapeutic properties.
Formation of ε-PL Derivatives
Polymers and Copolymers: ε-PL can be polymerized or copolymerized with other monomers to create new materials with tailored properties. For example, ε-PL copolymers can be designed to have specific release profiles or enhanced stability in different environments.
Cross-Linking: Cross-linking ε-PL with other polymers or molecules can improve its structural stability and functional properties. Cross-linked ε-PL networks are used in applications such as controlled drug release and tissue engineering.
Physical and Chemical Modifications
Encapsulation Techniques
Microencapsulation: Encapsulating ε-PL in microcapsules can protect it from environmental factors and control its release. This technique is used in pharmaceuticals and food applications to enhance stability and efficacy.
Nanoparticle Formation: Forming nanoparticles with ε-PL can improve its delivery and bioavailability. ε-PL nanoparticles are employed in targeted drug delivery and diagnostic applications.
pH and Temperature Stability
Stabilization Techniques: Modifying ε-PL to enhance its stability under varying pH and temperature conditions can extend its shelf life and effectiveness. Techniques include chemical modifications and the use of stabilizing agents.
Functional Modifications
Enhanced Antimicrobial Activity
Activity Enhancement: Structural modifications can increase the antimicrobial activity of ε-PL against specific pathogens. For example, modifications that improve ε-PL’s interaction with microbial membranes can enhance its effectiveness.
Biocompatibility and Safety
Biocompatibility Improvements: Modifying ε-PL to improve its biocompatibility is crucial for pharmaceutical and medical applications. Ensuring that modified ε-PL does not induce adverse immune responses or toxicity is a key consideration.
Emerging Applications of Modified ε-Polylysine Hydrochloride
Pharmaceuticals
Controlled Drug Delivery Systems
Modified ε-PL is increasingly used in controlled drug delivery systems due to its ability to form stable complexes with drugs and release them in a controlled manner. Applications include:
Targeted Drug Delivery: ε-PL-based nanoparticles or conjugates can deliver drugs specifically to target tissues or cells, improving therapeutic efficacy and reducing side effects.
Sustained Release Formulations: ε-PL’s modification can enable the development of sustained release formulations that provide a steady release of drugs over time.
Gene Delivery
ε-PL’s ability to form complexes with nucleic acids makes it a promising candidate for gene delivery applications. Modified ε-PL can be used to deliver therapeutic genes or RNA molecules to specific cells, offering potential treatments for genetic disorders and cancers.
Food Industry
Preservatives and Antimicrobials
Modified ε-PL with enhanced antimicrobial properties is used as a preservative in food products. Its effectiveness in preventing microbial contamination and spoilage makes it valuable for extending the shelf life of food items.
Edible Coatings
ε-PL-based edible coatings are being developed to improve the quality and shelf life of food products. These coatings can provide antimicrobial protection while being safe for consumption.
Cosmetics
Anti-Aging and Skin Care Products
Modified ε-PL is used in cosmetics for its antimicrobial and skin-conditioning properties. It can be incorporated into anti-aging creams, moisturizers, and other skincare products to enhance their efficacy and safety.
Wound Healing
ε-PL-based formulations are employed in wound healing products due to their antimicrobial activity and biocompatibility. Modified ε-PL can promote faster healing and reduce the risk of infection in wounds and burns.
Challenges and Future Directions
Scalability and Cost
Scaling up the production of modified ε-PL from laboratory to industrial scale poses challenges related to cost, consistency, and process optimization. Addressing these challenges requires advancements in production technology and efficient process scaling strategies.
Regulatory Considerations
Modified ε-PL must comply with regulatory requirements for safety, efficacy, and quality. Navigating the regulatory landscape for new ε-PL formulations and applications involves thorough testing and documentation to meet the standards set by agencies such as the FDA and EMA.
Research and Development
Ongoing research is essential for exploring new modifications and applications of ε-PL. Collaborative efforts between researchers, industry, and regulatory bodies can drive innovation and facilitate the development of advanced ε-PL-based products.
Sustainability
Developing sustainable production methods and utilizing renewable resources are important considerations for the future of ε-PL production. Emphasizing environmental impact reduction and resource efficiency aligns with global sustainability goals.
Conclusion
Advancements in the synthesis and modification of ε-Polylysine hydrochloride are expanding its applications and enhancing its performance across various industries. Innovations in fermentation technology, structural modifications, and emerging applications highlight the potential of ε-PL in pharmaceuticals, food, and cosmetics.
While challenges such as scalability, cost, and regulatory compliance remain, ongoing research and technological developments offer promising solutions. The future of ε-PL lies in continued innovation, addressing sustainability concerns, and exploring new applications to meet the evolving needs of various industries. As research progresses, ε-PL is poised to play a significant role in advancing therapeutic, food safety, and cosmetic technologies.