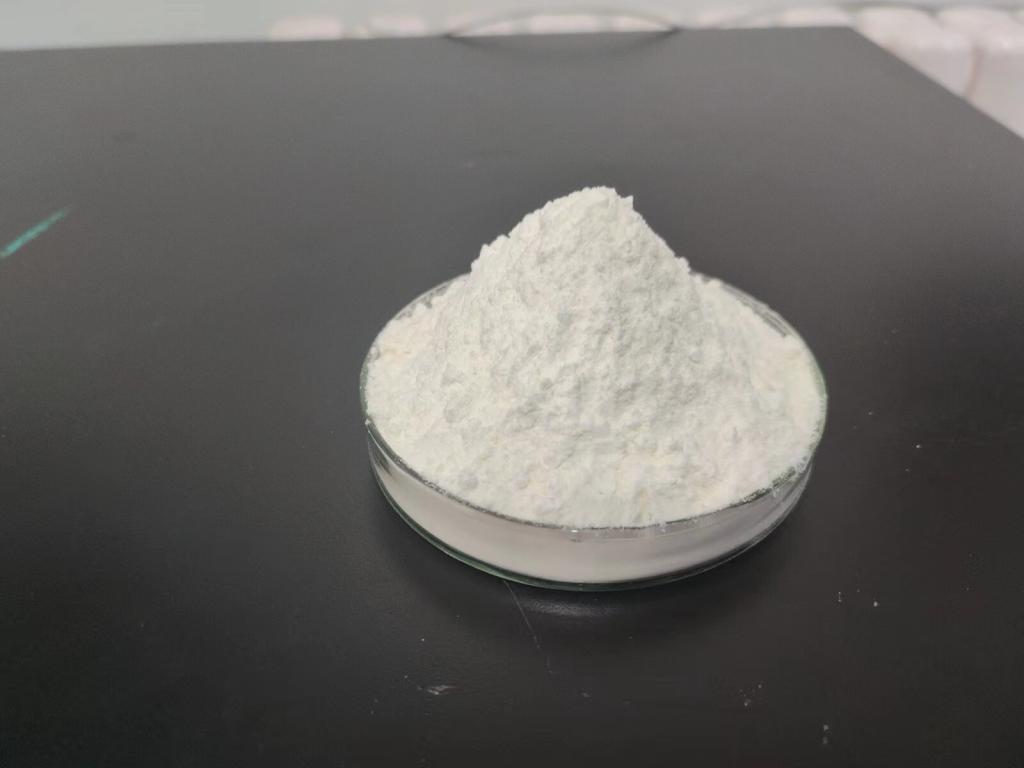
ε-Polylysine hydrochloride (ε-PL) is a natural antimicrobial peptide with broad-spectrum activity against a wide range of microorganisms. Due to its safety, efficacy, and biodegradability, ε-PL has gained attention as a food preservative and biomedical agent. However, the production and purification of ε-PL pose challenges due to its complex structure and low yields. This article explores recent advancements in production and purification methods for ε-PL, aiming to improve efficiency, yield, and quality.
Production of ε-Polylysine Hydrochloride:
The production of ε-PL involves fermentation processes using microbial strains capable of synthesizing ε-PL. Key advancements in ε-PL production methods include:
Microbial Fermentation: Streptomyces albulus is the most commonly used microorganism for ε-PL production. Advances in microbial fermentation techniques, such as strain selection, media optimization, and process control, have led to increased ε-PL yields and productivity. Genetic engineering approaches have also been employed to enhance ε-PL production by optimizing metabolic pathways and increasing precursor availability.
Substrate Utilization: Various carbon and nitrogen sources, including glucose, starch, soybean meal, and yeast extract, have been investigated as substrates for ε-PL production. Advances in substrate utilization strategies, such as feedstock selection, concentration optimization, and supplementation with amino acids, have improved ε-PL yields and reduced production costs.
Bioreactor Design: Advances in bioreactor design and operation have contributed to the scale-up of ε-PL production from laboratory to industrial scale. Bioreactors with controlled parameters, such as temperature, pH, dissolved oxygen, and agitation speed, optimize microbial growth and ε-PL synthesis, leading to improved productivity and efficiency.
Purification of ε-Polylysine Hydrochloride:
The purification of ε-PL from fermentation broth involves multiple steps to remove impurities and concentrate the desired product. Key advancements in ε-PL purification methods include:
Precipitation Techniques: Precipitation methods, such as ethanol precipitation, acetone precipitation, and salting-out, are commonly used for initial purification of ε-PL from fermentation broth. Advances in precipitation techniques, such as optimization of solvent concentration, temperature, and pH, improve ε-PL recovery and purity.
Chromatography: Chromatographic techniques, including ion exchange chromatography, size exclusion chromatography, and reversed-phase chromatography, are employed for further purification of ε-PL. Advances in chromatographic methods, such as column design, stationary phase selection, and elution conditions, enhance ε-PL separation efficiency and yield.
Membrane Filtration: Membrane filtration techniques, such as ultrafiltration and diafiltration, are used for concentration and purification of ε-PL. Advances in membrane technology, such as membrane material, pore size, and operating conditions, optimize ε-PL recovery and purity while minimizing product loss.
Quality Control and Characterization:
Quality control and characterization of ε-PL are essential to ensure product quality, consistency, and safety. Key advancements in quality control and characterization methods include:
Analytical Techniques: Analytical techniques, such as high-performance liquid chromatography (HPLC), capillary electrophoresis (CE), and mass spectrometry (MS), are used to quantify ε-PL content, purity, and molecular weight distribution. Advances in analytical methods, such as method sensitivity, selectivity, and reproducibility, improve ε-PL characterization and quality assessment.
Structural Analysis: Structural analysis techniques, such as nuclear magnetic resonance (NMR) spectroscopy, X-ray crystallography, and circular dichroism (CD) spectroscopy, elucidate the molecular structure and conformation of ε-PL. Advances in structural analysis methods provide insights into ε-PL functionality, stability, and interactions with other molecules.
Biological Assays: Biological assays, such as antimicrobial activity assays and cytotoxicity assays, evaluate the efficacy and safety of ε-PL for specific applications. Advances in biological assay development, such as standardized protocols, reference materials, and quality control measures, ensure accurate and reliable assessment of ε-PL performance.
Applications of ε-Polylysine Hydrochloride:
ε-Polylysine hydrochloride finds diverse applications in the food, pharmaceutical, and cosmetic industries due to its antimicrobial, antiviral, and preservative properties. Key applications of ε-PL include:
Food Preservation: ε-PL is used as a natural preservative in a wide range of food products, including meat, poultry, seafood, dairy, beverages, and bakery items. Its broad-spectrum antimicrobial activity inhibits the growth of spoilage organisms and pathogens, extending the shelf life of food products while ensuring safety and quality.
Biomedical Applications: ε-PL exhibits potential therapeutic properties, including antimicrobial, antiviral, antitumor, and immunomodulatory effects. It is being explored for applications in wound healing, drug delivery, tissue engineering, and oral hygiene products. Advances in ε-PL production and purification methods facilitate its development as a biomedical agent for various clinical applications.
Cosmetic Formulations: ε-PL is used in cosmetic formulations, such as skincare products, hair care products, and personal hygiene products, for its antimicrobial and preservative properties. It helps prevent microbial contamination and spoilage, extending the shelf life of cosmetic products while maintaining product safety and efficacy.
Future Perspectives:
Advancements in production and purification methods for ε-PL are expected to drive its widespread adoption in food, pharmaceutical, and cosmetic industries. Future research directions include:
Process Optimization: Continued optimization of fermentation, purification, and characterization processes to enhance ε-PL yield, purity, and quality.
Biotechnological Approaches: Exploration of biotechnological approaches, such as metabolic engineering, fermentation optimization, and enzyme engineering, to further improve ε-PL production efficiency and scalability.
Application Development: Expansion of ε-PL applications in emerging fields, such as biomedicine, agriculture, and environmental science, to harness its antimicrobial and therapeutic properties for diverse applications.
Sustainability Initiatives: Integration of sustainable practices, such as renewable feedstock utilization, waste valorization, and eco-friendly production processes, to minimize environmental impact and promote sustainable ε-PL production.
Conclusion:
Advancements in production and purification methods for ε-Polylysine hydrochloride have facilitated its widespread adoption in food, pharmaceutical, and cosmetic industries. Continued innovation and research efforts are expected to further enhance ε-PL production efficiency, yield, and quality, driving its application in diverse fields and contributing to sustainable development and improved human health.